Dr. Johan Christensen, IMDEA Materials Institute
Imagine being on your way to a well-deserved summer vacation when suddenly you collide with another vehicle. Fortunately, the chassis of your car deforms in a controlled and programmed manner upon impact, creating a safe zone for the occupants to emerge unscathed. Magic?
No, it’s not magic. It’s one possible future that we have to look forward to thanks to the so-called topological materials, the emerging field in metamaterial research.
Transformer cars, invisibility cloaks and silent cities are just three examples of what is to come.
Metamaterials: What are they and where are they heading?
The study of metamaterials has opened new frontiers in recent years, allowing us to interact with, and control, sound and light waves in exotic ways that were previously inconceivable.
For example, there are the well-known metamaterial coatings that can absorb or redirect light waves. This prevents light from reflecting off the object, making it indistinguishable to the human eye, creating a kind of “invisibility cloak.”
Some metamaterials have also been designed to induce a negative refractive index, a phenomenon never seen in natural materials, where light or sound waves bend in the opposite direction. This concept of negative refraction has fascinating implications, such as creating the perfect lens with optical properties far superior to current ones.
How a donut can transform into a coffee cup
This may sound like science fiction to many, but within the scientific community, this research has been ongoing for over two decades.
Now, researchers are focusing their attention on topological materials, a type of metamaterial that remains robust regardless of the applied level of deformation. The most common example used to illustrate this concept is that of a donut that can be continuously transformed into a coffee cup since they are topologically equivalent. In other words, they can be reshaped into each other without damaging or changing the fundamental properties of either object.
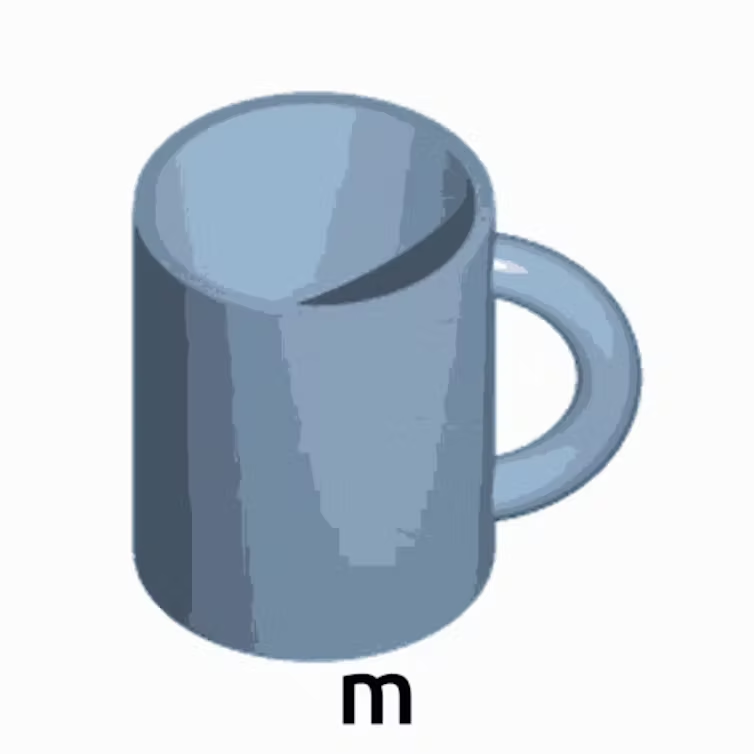
This topological robustness has been extensively studied in the field of condensed matter physics and has led to unusual electronic states where materials become insulating on the inside and conducting on the outside, known as topological insulators, first observed in 2007. These unique conditions can lead to waveguides immune to defects and disorders, meaning that light and sound waves can move freely without being affected by structural defects or manufacturing imperfections. This has promising applications today in transistors for quantum computing, as well as advanced magnetoelectronic and optoelectronic devices.
The outcome of an international collaboration between researchers from Switzerland, China, and the IMDEA Materials Institute in Spain, recently published in the journal Nature, highlights how topological degrees of freedom based on symmetries offer an alternative and unprecedented approach to the manipulation of light and sound, leading to new photonic and acoustic structures beyond the realm of traditional metamaterials.
Capturing light waves and defects by design
Today’s experts in metamaterials focus not only on optics but also on other areas of wave physics, such as acoustics.
This has a wide range of applications. For example, we could mitigate acoustic pollution around highways or in cities. We could even create surfaces that do not reflect sound or radar waves at all or offer different reflections from different directions. This could be incredibly important for stealthier vehicles in military applications. We are also exploring how such intentionally designed defects in the material itself, using 3D printing technology, can trap and/or manipulate light, acoustic, or electromagnetic waves.
In materials science, it is common to conduct experiments to study how deformations affect the structure and mechanical properties. This way, we can understand how a pre-designed and manufactured material will behave under the conditions being tested.
What we seek to achieve now and in the future is to go a step further. In fact, we will not only be able to understand how a material will deform, but we will be able to design that deformation within the material itself. In summary, we can create defects by design.
In automobile manufacturing, “safe zones” could be created, as in the previous example, where vehicle components will be designed in such a way that the deformation of the chassis occurs in a manner that maximizes the safety of the driver. In the aerospace industry, parts can be designed in such a way that, in the event of a failure, the damage to nearby components or the overall integrity of the structure can be controlled in a pre-established manner.
Although this research is still in a conceptual phase, it paves the way for the construction of the future.
<img src=”https://counter.theconversation.com/content/208552/count.gif?distributor=republish-lightbox-advanced” alt=”The Conversation” width=”1″ height=”1″ style=”border: none !important; box-shadow: none !important; margin: 0 !important; max-height: 1px !important; max-width: 1px !important; min-height: 1px !important; min-width: 1px !important; opacity: 0 !important; outline: none !important; padding: 0 !important” referrerpolicy=”no-referrer-when-downgrade” />