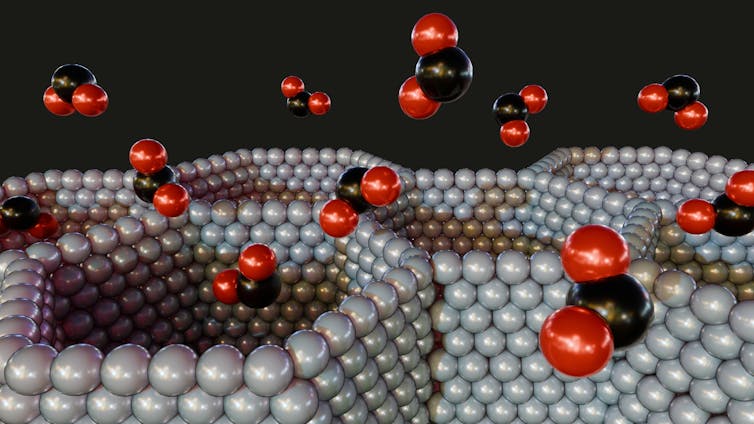
Representation of a porous MOF structure capturing carbon dioxide molecules. Love Employee/Shutterstock
Phuong Thúy Vo, IMDEA MATERIALES
From CO₂ capture to drug delivery, porous materials are becoming essential in a wide range of applications. Among them are the so-called metal-organic frameworks or MOFs, a type of crystal derived from nanotechnology, highly porous and formed by the union of metallic atoms and organic molecules.
A teaspoon of these crystals could cover the surface of an entire football field. Their adjustable structure and vast internal surface make them particularly promising in areas such as gas storage and catalysis.
However, there is still a significant gap in our understanding of the interfaces of MOFs within compounds, that is, the boundaries between the metallic and organic parts. These are studied with software tools that are still somewhat limited in accurately characterising and discovering these unique and complex materials.
Inspired by nature
The usefulness of porous materials can be observed in our surroundings. In wood, the transport of water and nutrients from the roots to the leaves of a tree is made possible by its porous properties, which also provide greater strength and flexibility.
The porous structure of natural sponges allows them to filter water and extract nutrients. Similarly, porous materials like corals create habitats that support a wide variety of marine life. Meanwhile, porous rocks, such as limestone or dolomite, facilitate the accumulation and flow of fluids, including oil, water, and even natural gas, within their formation.
This ability to accumulate and distribute fluids within what would otherwise be a solid material is also one of the most relevant properties of porous materials in the human body. Bone is a key example of a natural porous material. Without this essential feature, the flow of blood and nutrients that keeps bones alive and healthy would not be possible.
Aerogels, Zeolites and MOFs
A material is considered porous when it contains small holes or empty spaces in its structure, known as pores. The size of these pores can range from nanometers to micrometres. Some of the most promising categories of porous materials are aerogels, zeolites, and MOFs.
- Aerogels: these are ultralight materials with extraordinary thermal insulation properties. In spacecraft, they protect the interior from cosmic cold. On Earth, they insulate our buildings, keeping them warm in winter and cool in summer.
- Zeolites: These are crystalline aluminosilicate minerals, fundamental chemical compounds due to their abundance, versatility, and multiple applications in construction, glass, ceramics, and various technological industries. They are widely used as commercial adsorbents and catalysts. Their porous structure, formed by a framework of silicon, aluminium, and oxygen atoms, acts as a sieve that filters the useful from the undesirable.
- MOFs: These are crystalline materials formed by the union of metal ions or groups with organic ligands. Their porous structure provides large surfaces for gas storage and separation, and they are valuable for their ability to selectively absorb gases in their pores.
These materials are very promising for transforming sectors ranging from energy storage to environmental recovery. However, they are complex, and their behaviour depends on the delicate relationship between the metallic and organic components.
The key role of interface engineering
In the field of material innovation, porous materials continue to revolutionise multiple industries due to their high specific surface area, adjustable pore structures, and exceptional adsorption properties. These characteristics make them highly valuable in applications such as gas and energy storage, as well as in carbon capture.
One of the most promising advances in this area is the integration of MOFs (metal-organic frameworks) with polymers, resulting in MOF-polymer composites. Polymers are large molecules formed by the union of simple units called monomers. The hybrid materials that result from combining these with MOF crystals combine the unparalleled adsorption and selectivity capabilities of MOFs with the flexibility and processability of polymers.
A crucial factor that determines the performance of these composites is the MOF-polymer interface. This region influences mechanical stability, thermal transport, and overall efficiency in real-world applications. Small variations in the structure of the interface can significantly affect macroscopic properties such as mechanical strength, ionic conductivity, and thermal resilience.
By optimising these interfaces, researchers can adjust the material’s behaviour to meet specific industrial demands. In recent years, work in this field has been particularly relevant in gas separation.
At the forefront of this research on MOF-polymer composites is the M4MID project, funded by the European Union’s Marie Skłodowska-Curie Actions (MSCA) and currently being carried out at IMDEA Materials Institute.
To gain deeper insights into interfacial phenomena, the IMDEA Materials team behind the project has developed an automated process to generate virtual interfacial structures within MOF-polymer composites. These computational models allow researchers to explore interfacial interactions, simulate vibrational behaviour, and analyse heat transfer mechanisms.
By making modifications to the interfaces, the team has demonstrated that even small adjustments can lead to significant changes in the material’s properties, from enhanced mechanical strength to improved thermal conductivity.
These findings highlight the crucial role of interface engineering in optimising MOF-polymer composites for high-performance applications. As the research progresses, the IMDEA team remains committed to refining these materials, ensuring their large-scale viability for clean energy development, environmental sustainability, and advanced technological solutions.
Phuong Thúy Vo, Postdoctoral Researcher in Computational Chemistry, IMDEA Materials.
This article was originally published in The Conversation. Read the original here.